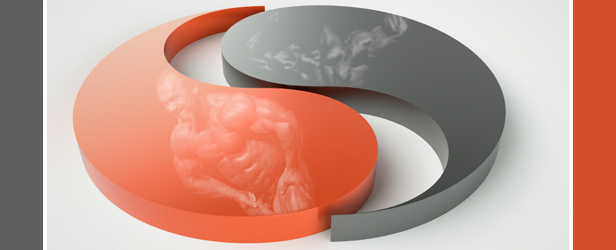
It happens to all of us at some point. We suffer an injury, take a long trip or vacation, or even experience true overtraining (1–3), leaving essentially no choice but to take a break from training. The cards are dealt and you’re handed the most dreaded of fates—the “D word” (detraining).
When it’s time to get back in the saddle, most iron addicts are itching to go full bore, “gung ho from the get go” to get back to previous strength, size, performance, and appearance. Long standing forms of linear periodization (e.g., Matveyev’s classic model [4, 5]) along with common sense suggest easing back with lighter loads (perhaps with higher training volume) to reverse off-season deconditioning. The coaches reading this have probably witnessed detraining in athletes after a long (sometimes lazy) off-season. Many of you may also be personal trainers who have “seasonal” clients or those who “fall off the wagon” due to work, finances, or even snow birding. Trainees return to you in a state of training far different than the last time you worked with them.
Naturally, the principle of reversibility applies here: Your gains will be reversed when the stimulus of adaptation is lost (6, 7). But really, aside from a bruise to the ego, how much does one lose in only a few weeks or months after training for years? Are losses greater or more rapid in the highly trained or more so in relative newbies to the iron game? How fast do gains come when starting back in the gym? Is it necessary to blast full bore into one’s previous training schedule and simply suffer with extreme soreness and (potentially dangerous [8]) muscle damage? I think that we’ve all heard and/or seen the horror stories—personal trainers pushing clients far beyond what’s necessary for a first training session followed by a predictable no-show for the next appointment. (This obviously isn't necessary or good for business.)
In the beginning
It’s commonly understood (and supported with research) that the initial, relatively rapid gains in strength with a novel weight training regime are primarily neurological in nature (9, 10). Maximal muscle electrical activity (indicative of neural activation) increases rapidly, substantially, and in parallel with strength gains (11, 12) and to a greater extent than muscle cross-sectional area (13).
On the other hand, muscle remodeling does indeed begin from the first workout. (Ever been surprised how sore you were from something really “easy” after a layoff?) It just takes one bout to stimulate contractile protein and collagen synthesis (14). In fact, type II muscle fiber type interconversions and strength gains are quantifiable within two weeks of training onset (15, 16). One study has even found significant increases in muscle thickness after only two training sessions (17). Heck, only twenty-four (twice daily for six days/week) blood flow restriction training sessions spread out over two weeks produced increases in muscle volume of 8–10 percent (18). (These kinds of gains, even when spread out over two months, are substantial and visibly noticeable (19, 20).)
Something else pretty cool happens when muscle grows from repeatedly banging away in the gym. Because skeletal muscle fibers are relatively large, they must be populated by many nuclei, hypothetically such that each nucleus governs a relatively consistent volume of the cell, otherwise known as its “myonuclear domain" (21, 22). Thus, as muscle fibers enlarge, nuclei are typically added as satellite cells (23) sandwiched between regular muscle fibers (24) and undergo division and donate nuclear (genetic) material. This maintains myonuclear domain size and keeps order in the growing fibers (25, 26). In fact, myonuclei may be added even before the muscle cell begins to get bigger (27), underscoring the importance of even distribution of these localized “genetic outposts” during the hypertrophic remodeling process (28). This is a major restructuring of the muscle cell of course. While a short period of training (one to three months) may cause muscle satellite cells to proliferate (increase in number), if training stops, this extra nuclear material never makes it into the fibers themselves as actual myonuclei (29). (Make note of this, as these satellite cells seem to be intimately involved in the phenomenon of “muscle memory,” discussed in Part II of this article series.)
The deload—no big worries
In my experience coaching and training clients, when the structure of a deloading period (several days up to a couple weeks) is left to the individual, loss of strength is highly variable. Some clients come back refreshed and stronger whereas others have taken a step back, which is quickly regained.
This variability is likely dependent upon the frequency (30) and intensity (31–33) of whatever training takes place during the deload. The overreaching status of the trainee before the layoff probably plays a part as well (1, 2) not to mention diet and other lifestyle factors. An athlete who spends his deload week hitting the craps tables in Vegas might come back less refreshed (and weaker) than one who enjoys a mini-vacation barbequing and lounging by the pool.
It seems plausible that just as the adaptation to training is variable but somewhat genetically determined (34), the results of detraining may vary similarly but also be predictable based on the initial state of training. Let’s take a closer look.
Strength detraining and jogging your muscle memory
Here you are after many weeks or months of real detraining. You’ve done nothing in the gym for a long while. Obviously, the mirror gives some indication of your relatively slovenly status, but the mind can play tricks as well. What does exercise science tell us to expect on the fateful first day back?
Some early research (in the 80s and 90s) indicated that the time course of strength and muscle size loss follows a somewhat similar (reversed) pattern during detraining (13, 35). For instance, a well respected group of Finnish researchers found that 50 percent of strength gained over sixteen weeks of training was lost in eight weeks of detraining (11).
A longer study of (quite brutal) training and detraining paints a more optimistic picture. Ohio University researchers set out to examine the concept of “muscle memory” in two dozen women who trained the lower body for twenty weeks (twice weekly performing 3 X 6–8 reps to failure of squats, leg presses, knee extensions, and hamstring curls). They roughly doubled strength and increased the size of their type II muscle fibers by about 50 percent (36). (By the way, skin folds dropped substantially and their thigh and gluteal circumferences were unchanged. I would be willing to bet that there were some pretty impressive transformations among these subjects.) Six of the women came back after seven to eight months of detraining to find that they’d only lost about 50 percent of their gains in strength, but size was preserved in the type I and IIA muscle fibers. (Only the type IIB fibers, which were likely not used regularly during many daily activities (37), atrophied significantly (38).) When these hardy half-dozen retrained, they rapidly recovered their gains in strength and fiber size in only six weeks.
A more recent study also found that strength gains after only nine weeks of training were preserved after about seven months of detraining in both the young and old (39). Other research has shown remarkable preservation of strength (but less so for power) in recreationally trained men who discontinued their usual routines for six weeks (40). Also, recently acquired eccentric strength may be better preserved than concentric strength (41), perhaps because the ability to disinhibit force production (force production is inhibited neurologically during eccentric actions [42]) is better retained (43, 44) during detraining.
Keep some of it
So it seems that the “use it or lose it” adage is not entirely true when it comes to muscle strength and size. In Part II of this article, we’ll take a closer look at “muscle memory” and how it is that years in the weight room can pay long-term dividends even after taking a prolonged break from heavy lifting.
References
- Halson SL, Jeukendrup AE (2004) Does overtraining exist? An analysis of overreaching and overtraining research. Sports Medicine 34(14):967–81.
- Urhausen A, Kindermann W (2002) Diagnosis of overtraining: what tools do we have? Sports Medicine 32(2):95–102.
- Vogel R (2001) Übertraining: Begriffsklärungen, ätiologische Hypothesen, aktuelle Trends und methodische Limiten. Schweizerische Zeitschrift für Sportmedizin und Sporttraumatologie 49(4)154–62.
- Stone MH, et al (1982) A Theoretical Model of Strength Training. Strength & Conditioning Journal 4(4):36–9.
- Matveev LP (1972) Periodisierung des sportlichen Trainings. Bartels & Wernitz.
- Timothy RH. "Detraining." In: Rippe J (2012) Encyclopedia of Lifestyle Medicine and Health. SAGE Publications, Inc: Thousand Oaks, 307–09.
- Urso ML (2009) Disuse atrophy of human skeletal muscle: cell signaling and potential interventions. Med Sci Sports Exerc 41(10):1860–8.
- Szczepanik ME, et al (2014) Exertional rhabdomyolysis: identification and evaluation of the athlete at risk for recurrence. Curr Sports Med Rep 13(2):113–9.
- Jones DA, et al (1989) Physiological changes in skeletal muscle as a result of strength training. Q J Exp Physiol 74(3):233–56.
- Blazevich AJ (2006) Effects of physical training and detraining, immobilization, growth and aging on human fascicle geometry. Sports Med 36(12):1003–17.
- Hakkinen K, Komi PV (1983) Electromyographic changes during strength training and detraining. Medicine & Science in Sports & Exercise 15(6):455–60.
- Moritani T, deVries HA (1979), Neural factors versus hypertrophy in the time course of muscle strength gain. Am J Phys Med 58(3):115–30.
- Narici MV, et al (1989) Changes in force, cross-sectional area and neural activation during strength training and detraining of the human quadriceps. Eur J Appl Physiol Occup Physiol 59(4):310–9.
- Moore DR, et al (2005) Myofibrillar and collagen protein synthesis in human skeletal muscle in young men after maximal shortening and lengthening contractions. Am J Physiol Endocrinol Metab 288(6):E1153–9.
- Staron RS, et al (1994) Skeletal muscle adaptations during early phase of heavy-resistance training in men and women. J Appl Physiol 76(3):1247–55.
- Abe T, et al (2000) Time course for strength and muscle thickness changes following upper and lower body resistance training in men and women. Eur J Appl Physiol 81(3): 174–80.
- DeFreitas JM, et al (2011) An examination of the time course of training-induced skeletal muscle hypertrophy. Eur J Appl Physiol 111(11):2785–90.
- Abe T, et al (2005) Skeletal muscle size and circulating IGF-1 are increased after two weeks of twice daily Kaatsu resistance training. Int J Kaatsu Training Res 1:6–12.
- Ruther CL, et al (1995) Hypertrophy, resistance training, and the nature of skeletal muscle activation. J Strength Cond Res 9:155–59.
- Stevenson SW, Dudley GA (2001) Dietary creatine supplementation and muscular adaptation to resistive overload. Medicine and Science in Sports and Exercise 33(8):1304–10.
- Allen DL, et al (1999) Myonuclear domains in muscle adaptation and disease. Muscle Nerve 22(10):1350–60.
- Pallafacchina G, et al (2013) Role of satellite cells in muscle growth and maintenance of muscle mass. Nutrition, Metabolism and Cardiovascular Diseases 23(S1):S12–18.
- McCarthy JJ, et al (2011) Effective fiber hypertrophy in satellite cell-depleted skeletal muscle. Development 138(17):3657–66.
- Wang YX, Rudnicki MA (2012) Satellite cells, the engines of muscle repair. Nat Rev Mol Cell Biol 13(2):127–33.
- Petrella JK, et al (2006) Efficacy of myonuclear addition may explain differential myofiber growth among resistance-trained young and older men and women. Am J Physiol Endocrinol Metab 291(5):E937–46.
- Bamman MM, et al (2007) Cluster analysis tests the importance of myogenic gene expression during myofiber hypertrophy in humans. J Appl Physiol 102(6):2232–9.
- Bruusgaard JC, et al (2010) Myonuclei acquired by overload exercise precede hypertrophy and are not lost on detraining. Proc Natl Acad Sci U S A 107(34):15111–6.
- Petrella JK, et al (2008) Potent myofiber hypertrophy during resistance training in humans is associated with satellite cell-mediated myonuclear addition: a cluster analysis. J Appl Physiol 104(6):1736–42.
- Kadi F, et al (2004) The effects of heavy resistance training and detraining on satellite cells in human skeletal muscles. J Physiol 558(Pt3):1005–12.
- Bickel CS, et al (2011) Exercise dosing to retain resistance training adaptations in young and older adults. Medicine and Science in Sports and Exercise 43(7):1177–87.
- Mujika I (2010) Intense training: The key to optimal performance before and during the taper. Scand J Med Sci Sports 20(S2):24–31.
- Gibala MJ, et al (1994) The effects of tapering on strength performance in trained athletes. International Journal of Sports Medicine 15(8):492–7.
- Izquierdo M, et al (2007) Detraining and tapering effects on hormonal responses and strength performance. Journal of Strength and Conditioning Research/National Strength & Conditioning Association 21(3):768–75.
- Timmons JA (2011) Variability in training-induced skeletal muscle adaptation. J Appl Physiol 110(3):846–53.
- Colliander EB, Tesch PA (1992) Effects of detraining following short term resistance training on eccentric and concentric muscle strength. Acta Physiol Scand 144(1):23–9.
- Staron RS, et al (1990) Muscle hypertrophy and fast fiber type conversions in heavy resistance-trained women. Eur J Appl Physiol Occup Physiol. 60(1):71–9.
- McComas AJ (1996) Skeletal muscle: Form and function. Champaign, IL: Human Kinetics.
- Staron RS, et al (1991) Strength and skeletal muscle adaptations in heavy-resistance-trained women after detraining and retraining. J Appl Physiol 70(2):631–40.
- Lemmer JT, et al (2000) Age and gender responses to strength training and detraining. Med Sci Sports Exerc 32(8):1505–12.
- Kraemer WJ. et al (2002) Detraining produces minimal changes in physical performance and hormonal variables in recreationally strength-trained men. J Strength Cond Res 16(3):373–82.
- Andersen LL, et al (2005) Neuromuscular adaptations to detraining following resistance training in previously untrained subjects. Eur J Appl Physiol 93(5–6):511–8.
- Dudley GA, et al (1990) Effect of voluntary vs. artificial activation on the relationship of muscle torque to speed. Journal of Applied Physiology 69(6):2215–21.
- Sale DG (1988) Neural adaptation to resistance training. Med Sci Sports Exerc 20(5S):S135–45.
- Latella C, et al (2012) Reduction in corticospinal inhibition in the trained and untrained limb following unilateral leg strength training. Eur J Appl Physiol 112(8):3097–107.