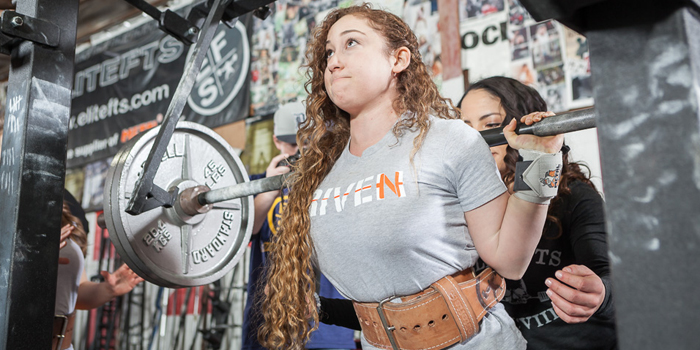
Did you know that muscle is an organ that has one of the lowest levels of protein synthesis in the entire body? Crazy and slightly disappointing, right? Here is the good news: It also loves to adapt. So, when you give it a stimulus that requires it to grow, in most conditions (and as long as you don’t do a bunch of counterproductive stuff), it will. However, that adaptability is two-sided, and so when given a stimulus not to grow, like stopping lifting and sitting on the sofa as if you were a bed rest patient, it will also adapt by decreasing its muscle volume (but not nuclei per se).
With this knowledge, consider yourself #woke (kidding) and realize that your program and what you do in the gym greatly dictates the gains you make. Realize that if you don’t challenge it (aka goof off in the weight room every day and move the same weights sets and reps), it won’t grow. Realize that if you train smart and start to look at training sessions as sessions of muscle challenge (and give your muscles sufficient recovery), you will probably begin to be way more efficient at progressing in the gym.
RECENT: How to Identify the Real-Deal Experts
To really do that, you need to understand muscle and what adaptations happen when you train. And I don’t just mean the obvious answer of your muscles getting bigger; I mean what happens from a 10,000-foot to a nanometer perspective.
Muscles’ Make-Up
Muscle is highly adaptable. This is why competitive lifters are often seen adding more and more mass over their careers (1), whereas people who are injured and/or immobilized experience reductions in muscle mass (2, 3). It's even why those presumed wives’ tales of high heels causing your calves to shorten are actually, in part, true (4). Muscle is one of the most plastic organs in the body, so if you want to understand its adaptability, you must first understand its make up.
Muscle is made up of a lot of stuff. On the smallest scale, small assemblies of proteins make up muscles’ force-producing units, which as known as sarcomeres. These force-producing units are made up of thick (myosin) and thin (actin) filament proteins, whose coordinated movements facilitate force generation within a muscle (5, 6). Different isoforms of the myosin heavy chain protein are what determine if a muscle is predominantly slow (type I) or a fast (type IIa, IIb) twitch muscle (7).
When a muscle contracts, myosin interlocks with actin at speeds that are myosin isoform-dependent. This is where the terms slow and fast twitch muscle fibers come from. When it comes to adaptation, fast fibers are most responsive to overload (8), whereas slow fibers are most responsive to underload (9). This makes a lot of sense, doesn’t it?
On a larger scale, these contractile units are strung together in a series, one after another, to form what is known as myofibrils. It is these myofibrils that make up about 80 percent of a muscle cell/fiber, as muscles are just filled fibers grouped together in bundles (10). While the number of sarcomeres added in a series dictates the length of a muscle, the addition of sarcomeres in parallel is what determines the cross-sectional area of a muscle. As you can see, what happens to the muscle on the smallest scale (in terms of sarcomere organization) has a drastic effect on muscle length and size, both of which ultimately mediate the potential of a muscle to generate force.
Why is That Important?
At the smallest scale when you challenge a muscle to lift more weight, it adapts to that demand, increasing in strength and size. But what’s really causing that increase? As mentioned above it’s via an increase in those force-producing sarcomeres.
How does that happen? Following overload, muscle undergoes an increase in protein synthesis, and that increase can create more protein that makes up sarcomeres, which then results in more of sarcomeres.
Increasing the number of these contractile units means you can contract with more force; therefore, you get stronger. But there is more. Lifting doesn’t just dictate that you add more of them; it also dictates the organization of how you add them. In response to increasing force output, the adaptation is to add them in parallel (side by side, if you look at a muscle cross-section), which increases the cross-sectional area of muscle.
Boom! How incredible is the body! That’s what’s going on when you ask the muscle to increase in size.
READ MORE: Make it More Than Just a Warm-Up
However, not all things you do in the gym elicit that specific adaptation in muscle. For example, eccentrics are sometimes thought of as something to help you get through strength and/or size plateaus. But how does it differ from the gains in response to normal lifting? Sure, they make more damage, which promotes more repair and growth, but what else happens?
Eccentric loading has a hypertrophic effect that causes the muscle to lengthen and produce force, which means the adaptation can be the addition of sarcomeres in series and in parallel. This adaptation results in a longer, thicker fiber, and for some, a breakthrough in a plateau. This all matters because if you understand how training affects things on the sarcomeric level, you can easily program to break through plateaus.
Summing it Up
When it comes to getting bigger, it’s worth it to take a minute and understand how muscle adapts to training across scales. Why? Because if something ever breaks (i.e., your program isn’t working), this type of knowledge can help you outsmart your body and program into the promised land of new gains!
References
- D'Antona, G. et al. Skeletal muscle hypertrophy and structure and function of skeletal muscle fibres in male body builders. The Journal of physiology 570, 611-627 (2006).
- Campbell, E.L. et al. Skeletal muscle adaptations to physical inactivity and subsequent retraining in young men. Biogerontology 14, 247-259 (2013).
- Psatha, M. et al. A longitudinal MRI study of muscle atrophy during lower leg immobilization following ankle fracture. Journal of magnetic resonance imaging : JMRI 35, 686-695 (2012).
- Csapo, R., Maganaris, C.N., Seynnes, O.R. & Narici, M.V. On muscle, tendon and high heels. The Journal of experimental biology 213, 2582-2588 (2010).
- Huxley, A.F. & Niedergerke, R. Structural changes in muscle during contraction; interference microscopy of living muscle fibres. Nature 173, 971-973 (1954).
- Huxley, H. & Hanson, J. Changes in the cross-striations of muscle during contraction and stretch and their structural interpretation. Nature 173, 973-976 (1954).
- Caiozzo, V.J. Plasticity of skeletal muscle phenotype: mechanical consequences. Muscle & nerve 26, 740-768 (2002).
- Gregory, P., Low, R.B. & Stirewalt, W.S. Changes in skeletal-muscle myosin isoenzymes with hypertrophy and exercise. The Biochemical journal 238, 55-63 (1986).
- Thomason, D.B. & Booth, F.W. Atrophy of the soleus muscle by hindlimb unweighting. Journal of applied physiology 68, 1-12 (1990).
- Sherwood, L. Human physiology: from cells to systems.