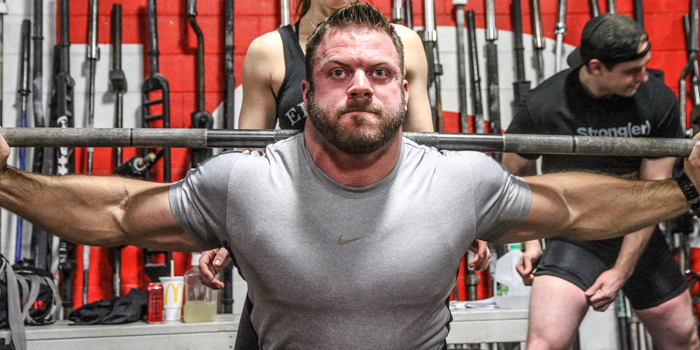
Lactate — a complex and misunderstood molecule. While the positive role of lactate has been propagated for the past 45 years thanks to the likes of George Brooks and others, it is still far too often demonized by many professors, coaches, and regular gym-goers. The history of the “lactic acid” phenomenon has been excellently described in previous literature, so this article will not focus on that area. Nor will this article devote much time to what lactate is not (the cause of soreness, fatigue, burning sensation, etc.), as this has already been written about extensively.
What this article will focus on is the role lactate plays within the body, whether this be in lactate's regenerative properties, acidic buffering abilities, positive role in the concussed and traumatic brain injured patients, a preferential fuel source for various bodily organs, or other characteristics.
RELATED: Misconceptions About Lactic Acid
A quick note on semantics. When calling lactate an entirely different molecule, such as lactic acid, it can be a problem. I dislike those who bring about issue with semantics and pedantism as much as the next person; however, lactate and lactic acid cannot and should not be interchangeable in one’s vernacular. A history of the lactic-acid era should be enough of a reason as to why this should not take place. Confusion and a further misunderstanding of lactate will inevitably come about. The synonymic portrayal of “acid” being “hurtful, wounding, venomous” can, in and of itself, lead the unknowing to view this metabolite in a poor light. While possibly causing issues, this area is rather trifling and deserves no further space within this article. I simply feel being as accurate as possible is the most advantageous path to understanding.
What Lactate Isn’t
Now, a brief description as to what lactate is not. A portion of this article will be dedicated to the metabolic role that lactate plays. Thus, greater detail within this section will not be given. Lactate accumulation does not cause (much) fatigue. It does not cause one to feel sore. Lactate is not a dead-end metabolite. It does not need to be “flushed out” the following day of a sporting event (the clearance rate of lactate following a specific event, while dependent upon the recovery modality put into place, is roughly 60-120 minutes. This being the case, accumulated lactate metabolites are simply a non-issue the following day). And lastly—possibly the most propagated misinterpretation of them all—lactate does not cause the “burning sensation” during a very challenging training session.
What Lactate Is
Lactate is a metabolite formulated via the enzyme lactate dehydrogenase (LDH) from pyruvate. This takes place as the last step of the glycolytic pathway (either glycolysis or glycogenolysis). It is a misnomer to differentiate or separate the divergent pathways following either glycolysis or glycogenolysis being due to insufficient oxygen levels in relation to oxidative-phosphorylation. Oftentimes, glycolysis is considered anaerobic or aerobic glycolysis dependent upon whether pyruvate is taken up into the mitochondria or pyruvate is catalyzed into lactate. This, however, is an incorrect notion. Lactate is produced at all times — during rest, moderate activity, among other activities with ample oxygen supply. This being so, the phrasing of “fast” or “slow” glycolysis is the most accurate description. As L.B Gladden describes, “The connection to increasing lactate production and higher muscle and blood lactate is that the requisite increase in ([ADP][Pi]/[ATP]), to compensate for the lower intramuscular partial oxygen pressure (iPO2), is a potent stimulate of glycolysis”. Thus, a reduction in the available oxygen, while possible to stimulate “fast” glycolysis and inevitably an increase in lactate production, is only one avenue of which increases the total lactate production.
Lactate: A Buffering Metabolite
Lactate is often thought of, as previously mentioned, as the primary culprit in relation to the onset of fatigue. Not only is this (partially) incorrect, but it is quite the opposite. In actuality, lactate delays various aspects of fatigue. Taking a look at the biochemical nature of the enzyme LDH and its role in catalyzing pyruvate to lactate, it can be seen that the LDH process produces NAD+ which allows a key step within the glycolytic pathway to take place: the glyceraldehyde 3-phosphate dehydrogenase reaction. Along with this, through the catalyzing process of pyruvate to a lactate and NAD+, one proton molecule is consumed. This then mitigates the proton accumulation and subsequent drop in pH. This being the case, the process of producing lactate has an alkalizing effect and assists in the prevention of a decreased physiologic pH.
Additional benefits of the LDH reaction can be seen with the transportation of lactate to surrounding tissue to be oxidized along with its transportation to various organs such as the heart, liver, kidney, and the brain as a preferential means of fuel via gluconeogenesis. This is made assessable by the monocarboxylate transporter (MCT). MCT comes in various isoforms dependent upon the tissue-type within the body. However, looking specifically at the skeletal muscle, MCT1 is predominantly found in the oxidative/ST muscle fibers while MCT4 is preferentially located in the glycolytic/FT muscle fibers. The importance of MCT concerning the consumption of a proton is the fact that it is a symport, which allows for the transportation of two molecules — in this case a lactate and a proton. So, a proton is taken up during the LDH catalyzing process along with the lactate transportation process leading to an alkalizing effect and mitigation of a pH drop.
Lactate Accumulation: Eyes Into Fatigue
Now, if the ability of the body to utilize the lactate that is produced becomes too great, this lactate subsequently accumulates at a rapid rate and this period is considered the lactate threshold. The lactate threshold, while not the predominant cause of fatigue, can be a good indicator of the intermuscular and intramuscular environment in relation to various metabolite accumulation properties due to insufficient oxidative properties and thus an onset of fatigue within the muscle.
Furthermore, when looking at lactate as the culprit in relation to being the primary propagator of fatigue, I feel most individuals look at it in an either black or white perspective; it either causes fatigue or it doesn’t. I say this because, while lactate may not play the primary role during a fatiguing bout along with not playing a role in the “burning” sensation, soreness, etc., it has been shown that it may possibly negatively impact calcium kinetics via the decreased efflux of calcium from the sarcoplasmic reticulum leading to decreased contraction capabilities (15%) and tension development independent of the physiological pH.
A quick side-note: calcium “unmasks” the myosin globular head binding site via the movement of tropomyosin on the actin allowing for activity between actin and myosin allowing the filaments to slide along each other and muscular contraction to ensue. If calcium is released in reduced quantities, the ability for myosin and actin to interact is reduced.
Continuing with lactate and fatigue, other studies on skinned fibers have reported dysfunctional contractile properties due to a heightened state of lactate albeit at a lesser degree (5%). This demonstrates the importance of letting go of any bias, at least in relation to lactate. Is it the primary cause of fatigue? No. Might the accumulation of lactate during intensive bouts of training play a background role? Yes.
While taking this into consideration, it must be understood that the goal is to not decrease the lactate that is produced, as can oftentimes be seen with many supplements that voice the necessity to decrease lactate production. This will actually impede performance. The goal is to equip the body with the necessary tools to utilize the lactate that is produced, such as various LDH isoforms, MTC isoforms, the quantity of mitochondria, etc.
George Brooks demonstrated that high-level athletes do not produce less lactate; they actually produce greater quantities of lactate, while their body's ability to utilize the lactate produced is much greater than the average athlete. In trained cyclists, it was demonstrated that their lactate flux rates were greater than their untrained counterparts along with their maximal clearance rates also being greater. Thus, higher-level athletes produce greater levels of lactate. They are simply capable of utilizing this lactate. This is where the specificity of training is very important in relation to lactate production, transportation, and utilization. For example, it was recently indicated that lactate uptake/utilization by surrounding tissues and organs (active oxidative muscle fibers, liver, kidney, heart, lungs, brain) were shown to be directly dependent on lactate delivery via the MCT. MCT has been shown to double in quantity following chronic endurance training. Along with this, the necessity of training for improvement in lactate kinetics has been shown with a change of the LDH-isoenzyme. H-LDH has been shown to shift to an M-LDH isoenzyme following training, which plays a greater role in the oxidation of lactate to pyruvate. So, while the accumulation of lactate is certainly less than advantageous, it does not mean that lactate production in a whole is a negative bodily function. As previously mentioned, equipping oneself with the proper tools allows lactate to be used in the most effective manner and improve performance.
Lactate: A Signaling Molecule
Lactate has been shown to up-regulate over 600 genes in relation to mitochondria biogenesis and protein synthesis. Lactate has also been shown to upregulate brain-derived neurotrophic factor (BDNF), which stimulates the growth of neurons within the brain. Further, lactate stimulates a specific part of the brain called the locus coeruleus which is where much of the norepinephrine neurons are located. Upon stimulation, these neurons release norepinephrine allowing for greater brain function such as focus, motivation, appetite suppression, among others. The predominant way that lactate works as a signaling molecule is through signal transducing properties. In relation to mitochondrial biogenesis, lactate has been shown to upregulate the MAPK pathway with its secondary messenger processes taking place, affecting many different downstream signaling proteins.
As mentioned in the above paragraph, lactate upregulates over 600 genes. 673 to be precise. The predominant way that it does this is through an increase in both reactive oxygen species (ROS) and calcium (Ca2+). Following incubation of L6-type cells within lactate of either 10mM and 20mM, MCT1 (a lactate transporter) mRNA and PGC1-alpha (a mitochondrial biogenesis stimulator) mRNA were both upregulated relative to the non-incubated group. Similarly, looking at the whole muscle level of lactate-induced genetic expression change, it can be seen that MCT1, MCT4, CD147, COX, and LDH are all upregulated. Looking directly at the mitochondrial fractions, it was shown that MCT1, CD147, COX, and LDH were enhanced. Understanding what each of these proteins does is not necessary. The important thing is to understand that these proteins are necessary for proper metabolic functioning specifically dealing with lactate and the ability to take up and utilize the lactate that is produced.
RELATED: The Truth About Energy Systems
This biggest take away when looking at lactate's role as a signaling molecule is the necessity to incorporate various training interventions, which produce large quantities of lactate. While it is often propagated by those who find lactate to be “evil” to stay away from producing it, it is now apparent that to reap maximal adaptations, at least in relation to oxidative processes, occasional training regimens which produce high levels of lactate are paramount. Taking this into consideration, while football may be considered an alactic/aerobic sport in relation to the predominant energy systems utilized, diverging from this path and using workouts that do not specifically mimic the bioenergetic specificities of the sport may, at times, be warranted.
Lactate and Wound Healing
An often-overlooked subcategory of lactate is its role in wound healing and regeneration. In the presence of heightened lactate production in a given tissue that has been wounded, the collagen secretion of the surrounding fibroblasts has been shown to increase two-fold. Fibroblasts play a prominent role in remodeling fascial tissue. Increased lactate has been shown to increase transforming growth factor-B1 (TGF-B1). This enhanced TGF-B1 environment induces fibroblast and myofibroblast proliferation, which further increases the production and deposition of collagenous fibers. A possible explanation for this increased lactate production following injury and wound healing, as described by L.B Gladden, may be due to the increased leucocyte activity, which has been demonstrated as a key producer of lactate.
Furthermore, lactate increases the collagen precursor role which then upregulates procollagen mRNA production and subsequently collagen synthesis. Along with this, elevated lactate properties reverse the inhibitory role of ADP-ribosylation (a down-regulatory of collagen gene transcription in fibroblasts). This takes place by lactate accumulation forcing the conversion of NAD+ (a substrate for ADP-ribosylation) to NADH (not a substrate for ADP-ribosylation), which leads to a reduction in actionable ADP-ribosylation. Thus, elevated levels of lactate appear to be a key regulator of collagen synthesis and deposition. This is independent of the oxygen present; this being the case, a state of hypoxia is not necessary as was previously thought.
A similar yet different approach that lactate has on wound healing is through its role promoting new blood vessels to the site of injury. This can be seen through the enhanced vascular endothelial growth factor (VEGF) pathway within macrophages. This takes place via a similar process to the one mentioned above. ADP-ribosylation appears to reduce VEGF autocrine properties leading to a decreased synthesis of VEGF. Elevated lactate appears to alleviate the inhibitory role of ADP-ribosylation on VEGF leading to a greater blood supply to the site of injury. Furthermore, as described by Trabold and colleagues, lactate is a pH-independent vasodilator, which would allow for the increase of oxygen to the site of injury.
Taking all of this in, it is now evident that elevated levels of lactate concerning wound healing play a significant role in the regenerative process. Lactate accumulation at the site of injury upregulates procollagen mRNA transcription, collagen synthesis, collagen deposition, VEGF upregulation, and an enhanced oxygen supply.
Lactate: Gluconeogenesis and the Brain
The brain has uniquely high energy requirements. Approximately 20% of the oxygen and 25% of the glucose within the body is utilized in the brain. While this may be the case, the brain has very few energy reserves and subsequently relies on energy substrates from the circulation. The brain is an efficient organ and can utilize various forms of energy substrates such as glucose, pyruvate, glutamate, glutamine, and lactate. Lactate, however, has been observed as the preferential cerebral oxidative energy substrate (it’s also preferred in the skeletal muscle and heart). As a key energy substrate, lactate can be readily oxidized and utilized within both neurons and astrocytes due to shuttling processes between the mitochondria and the extracellular space. Of even greater interest, neurons, which possess high energy requirements, preferentially choose lactate over glucose when both substrates are present.
As a quick description of how this takes place, I will briefly describe the process to which lactate is taken up and used within the neuron. Glucose is the preferred substrate within the astrocyte. The pyruvate that is derived via this glycolytic pathway within the astrocyte is catalyzed to lactate via the LDH5-isoform and is transported to the neuron by means of the MTC1 and MTC4 lactate transporter. This lactate is then taken up by the neurons and is, through a gluconeogenic process, converted back to pyruvate by the LDH1-isoform. This is all made possible by intracellular and cell-to-cell lactate shuttles within the brain. Similar to skeletal muscle, neurons contain a mitochondrial lactate oxidation complex (mLOC), which allows for this shuttling process to take place. This is rather complex but incredibly interesting. Another example of lactate's important role within the body.
Lactate and the Concussed
Recently at UCLA, it was demonstrated that those suffering brain injuries take up and consume more lactate than at any other time. The same researchers showed that individuals with moderate to severe traumatic brain injury who were able to produce greater quantities of lactate recovered more quickly both physically and psychologically than their non-lactate producing counterparts. Following traumatic brain injury, along with the concussed, the brain's ability to break down sugar for glycolysis is impaired. The reasoning behind this is speculated to be due to:
- Greater glucose take-up from other parts of the body
- The relative complexity in relation to the enzymatic processes required before it can generate energy as compared to lactate.
Glucose is the primary fuel source for astrocytes, so they suffer along with the neurons, being that their primary fuel source is lactate, which is no longer produced in sufficient quantities. A current strategy to bypass this dysfunction within the brain for those individuals who are concussed along with those with TBI is to infuse lactate containing properties into the peripheral circulation, which will then be taken up by the brain via the blood-brain barrier and the MTC transporters and supply these neurons with its necessary fuel substrate. Specifically, in relation to those suffering from a concussion, as previously mentioned, a metabolic crisis within the brain takes place. The possibility of mitigating this metabolic crisis following the concussive injury would be incredibly beneficial and lactate infusion may be the key.
What Is Driving Fatigue?
So, we know that lactate is not the primary cause of fatigue. So what is? It is first important to understand that, while a great number of years and a number of studies have been dedicated toward this subject, we still do not fully understand exactly what is going on. I will briefly try to explain some of the leading theories in relation to the primary mechanisms of fatigue.
As previously mentioned, there is a myriad of mechanisms at play, both centrally and peripherally. Being that this article has predominantly been looking at the muscle, that is the area I will touch upon.
First off, lactate has been inappropriately called lactic acid for a reason. This reason is the correlation between lactate accumulation and hydrogen ion accumulation. Hydrogen ions are produced from various processes, but in this case, it is produced from the enzymatic pathway in relation to glycolysis. This increase in hydrogen ions while metabolic intensification increases lead to greater hydrolysis of ATP and subsequently greater hydrogen ion accumulation. This leads to an acidic environment and a decrease in pH. This decreased pH can cause issues with various contractile properties of the muscle, specifically calcium kinetics, as previously touched upon.
As a side note, this decrease in pH as a cause of fatigue is currently being debated, as some research demonstrates that it may not play a leading role. However, regardless of its direct role on immediate muscular contractile properties, the feedback mechanisms that take place would seem to decrease efferent stimulation to that specific muscle.
Furthermore, inorganic phosphate (Pi), the metabolite which is produced by means of the hydrolysis of creatine phosphate into creatine and Pi, has been a key contributor to the loss of contractile properties. More specifically, experiments on skinned fibers have shown to decrease force output in the presence of elevated Pi. The proposed explanation for this is that myoplasmic Pi appears to decrease force production by impacting cross-bridge function; specifically, this happens due to a reduced myofibrillar Ca2+ sensitivity. There are other areas where an increased state of Pi might induce dysfunctional contractile properties along with other parts of the body inducing fatigue, such as decreased propagation of the action potential, inhibited NA+/K+ pump leading to inadequate depolarization of the cellular membrane, subsequently further affecting the action potential, among many others. However, this is not an appropriate topic concerning the goal of this article.
Conclusion
Taking all of this in, we can see that not only is lactate not the predominate player in relation to fatigue, it actually plays a number of crucial roles in improving physical performance, from being a signaling molecule to buffering proton accumulation, to possibly preventing further damage to the brain following concussions. This area is continuing to be studied and new information regarding lactate is bound to come out. In the meantime, embrace lactate as your companion in the fight of fatigue. Not as the villain.
References
- Bélanger M., Allaman I., Magistretti P. J. Brain energy metabolism: focus on astrocyte-neuron metabolic cooperation. Cell Metabolism.
- Bouzier A.-K., Voisin P., Goodwin R., Canioni P., Merle M. (1998) Glucose and lactate metabolism in C6 glioma cells: evidence for the preferential utilization of lactate for cell oxidative metabolism. Dev. Neurosci.20:331–338
- Davies K. J. A., Packer L., Brooks G. A. (1981) Biochemical adaptation of mitochondria, muscle, and whole animal respiration to endurance training. Arch. Biochem. Biophys. 209:539–559
- Hashimoto T., Hussien R., Oommen S., Gohil K., and Brooks G. A.. 2007. Lactate sensitive transcription factor network in L6 cells: activation of MCT1 and mitochondrial biogenesis. FASEB J.21:2602–2612
- Tang F, Lane S, Korsak A, Paton JF, Gourine AV, Kasparov S et al. Lactate-mediated glia-neuronal signaling in the mammalian brain. Nat Commun 2014; 5: 3284.
Luke Olsen is a current graduate student within the Exercise Physiology department at the University of Kansas.