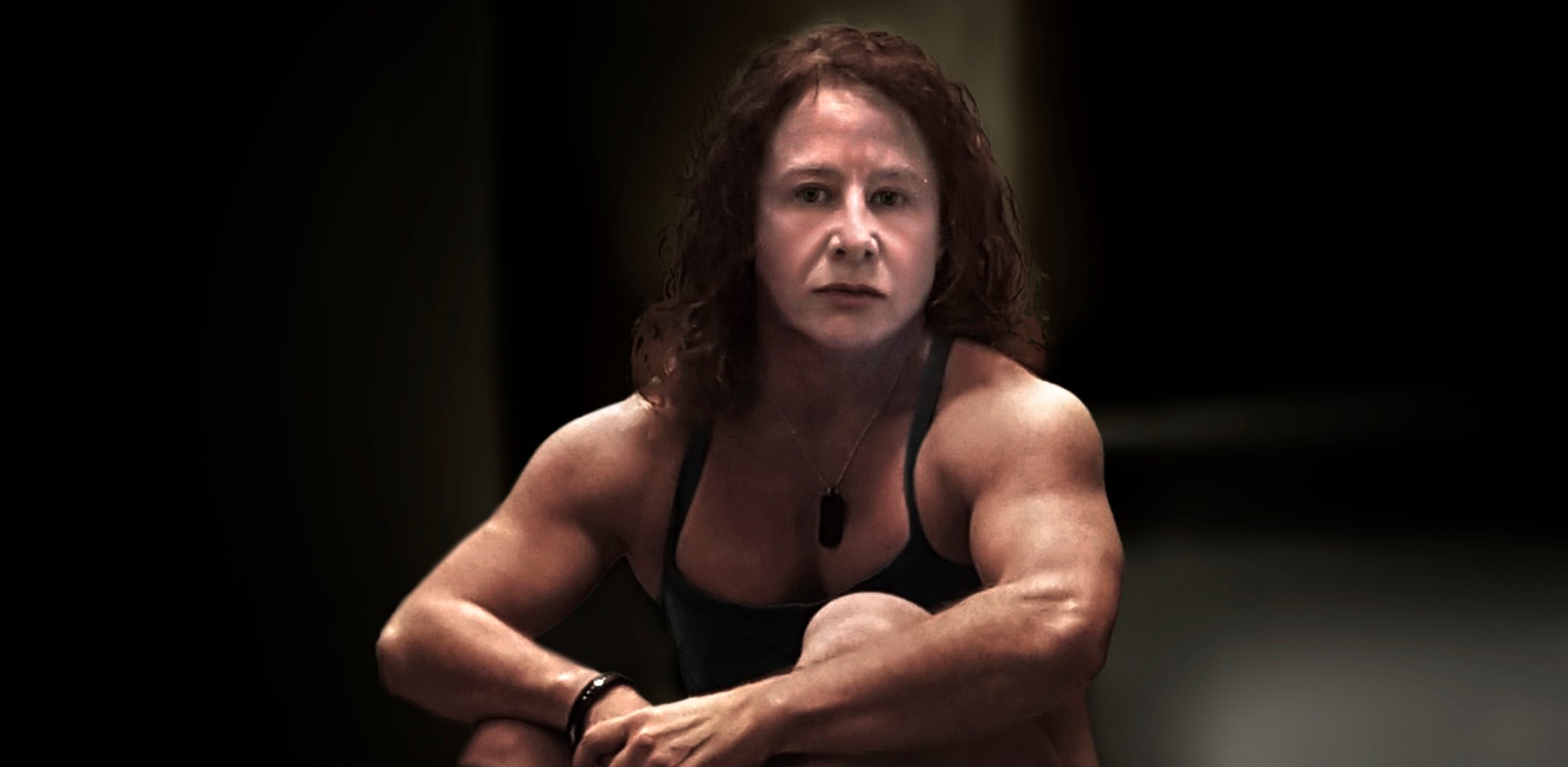
Introduction — Inter-individual Variation and Populational Diversity
Why do two athletes with the same bodyweight and similar training regime require different amounts of calories to maintain weight? Why do some people maintain or even gain performance on a very-low-carbohydrate diet while others lose it?
How much, in health and disease, in training and other aspects of life, is any rule applicable to all, to a subpopulation of humans or only one individual?
Why is something a sign of disease in some people and not in others?
In the nutrition sciences, after years of unsolved controversy concerning “the” best macro-nutrient diet composition, “the” best approach for weight loss or weight gain, “the” best parameters for a healthy weight or body compositions, cautious researchers are paying attention to relevant inter-individual differences. More than that, the inter-individual differences became a research topic and whether it is approached from an evolutionary, genetic, populational, ethnic or metabolomic perspective, most researchers and practitioners accept the need to understand diversity.
PART 1: One Training Size Fits One
Perhaps in a few years, every clinic in every corner of the world and even most citizens in industrialized countries will have their biomarker assessment mobile device, readings in plain language and easy to follow dietary instructions.
Meanwhile, read on to understand why we are so diverse and the ways to find your best diet options.
Epidemiological Patterns and Evolutionary Adaptations
Let’s start with milk, a common topic. Is non-human milk really a great food for everyone?
No, it is not. The chief reason why adult humans are intolerant to milk is the inability to digest lactose, a milk sugar. Humans need the enzyme lactase to do it. All infants have lactase but an estimated 65% of adults stop producing the enzyme as they stop breast-feeding.
Approximately 65% of the human population is lactose intolerant. Milk-protein allergies contribute to making non-human milk not a food option for 2/3 of humanity.
Understanding the distribution of lactose malabsorption is critical for the design of public health policies focused on nutrition or famine mitigation strategies (Storhaug et al 2017, Rangel et al 2016).
From: Storhaug et al 2017
From Rangel et al 2016
Cow milk protein allergies (CMPA) contribute to overall non-human milk intolerance. CMPA is the most common type of food allergy. Approximately 50% to 70% of subjects have skin reactions, 50% to 60% gastrointestinal symptoms and 20% to 30% have respiratory symptoms (Rangel et al 2016).
Two recent studies about the benefits of milk as a recovery drink for team athletes (Rankin et al 2018, Rankin et al 2020) were authored by scholars from the UK on local subjects. As was shown above, that is where cow milk intolerance is the lowest in the world.
Where does such significant variability come from? Evolution.
Lactase persistence can be directly observed by measuring the glucose concentration changes in the blood after milk ingestion or the amount of breath hydrogen, one of the products of gut bacteria metabolism of undigested lactose (Itan et al 2010).
From: Itan et al 2010
To make sense of the variation in digestive and metabolic traits we must examine human migration in the past 200,000 years, during which they were shaped by evolution. These include the digestibility of food components, like milk or starchy food and the ability to synthesize certain molecules, like long-chain fatty acids or vitamin D.
From James et al 2019
A global mapping model of human migration, based on the divergence of the mitochondrial DNA (which indicates the matrilineage). Timescale (ka) indicated by colors. CC BY-SA 3.0, from https://commons.wikimedia.org/w/index.php?curid=227326
There were several pre-historical migrations out of Africa. The evolutionary separation of modern chimpanzees and hominins ancestors happened 7-9 million years before present (YBP). Around 3-4 million YBP, the environment occupied by our pre-Homo ancestors changed from a forested one with an abundance of fruits to a drier environment where small and large animals could be collectively hunted, reinforcing social behavior. Roots and tubers were other sources of food. Major changes in dietary practices and digestive physiology occurred. These human ancestors ate much more protein, fat, and minerals. The fatty acid desaturase enzymes (FADS I and II) emerged at this time and allowed the oleic acid found in animals to be elongated to synthesize long-chain essential fatty acids. About two million YBP, some hominins migrated from Africa and along hundreds of thousands of years, colonized different areas in Europe and Asia. Neanderthals and Denisovans are two different species from this early stock.
The same new environmental conditions selected for a much larger brain, requiring more energy and more fatty acids for the enormous and convoluted organ.
It is assumed that Homo sapiens speciated around 200,000 YBP. Later, around 100,000 YBP, a sub-set of Homo sapiens migrated out of Africa and hybridized with archaic hominins from earlier diasporas. All non-African modern humans possess some Neanderthal ancestry and Melanesian populations additionally possess some Denisovan ancestry.
Humans spread and diversified. Linguistic studies have shown a correlation between four very different large African language groups and population DNA differences.
Genetic sub-types nutritionally adapted to different conditions continued to evolve: features adapted to pastoralism or agriculture, to colder and shorter-day environments or tropical ones were selected and retained.
Meanwhile, in Africa, archaic hominids continued to exist until about 35,000 YBP. Since then, hybridization with Homo sapiens provided that 2-5% of current African DNA derives from the ancient hominins. Homo sapiens colonized the diversity of African eco-systems and different digestive and dietary traits were selected.
From: James et al 2019
Despite thousands of years of genetic hybridization, differences consistent with earlier human genetic sub-populations still exist and determine much of present-day food intolerances, allergies, and human metabolism differences.
A "trellis" that emphasizes back-and-forth gene flow among geographic regions. By Source, Fair use, https://en.wikipedia.org/w/index.php?curid=36621737
The Fad Diets and the Anti-Fad-Fads: Things People Love to Hate
Fad diets are dietary programs promoted as optimal, universal, and aggressively marketed. It should be clear by now that none of them is scientifically consistent.
The easiest to discard is the “paleo diet”, promoted over the assumptions that present-day humans should eat as their “paleolithic” ancestors did. The Paleolithic period extends from the earliest known use of stone tools by hominins c. 3.3 million YBP, to the end of the Pleistocene c. 11,650 years before present. Down goes the paleo diet: there were multiple paleolithic ancestors, multiple diets, digestive and metabolic types. Still, the diet can and will be adequate for certain individuals, matching their digestive and metabolic makeup.
Now, the most controversial is the ketogenic diet (KD), a very low-carbohydrate, high-fat diet. Studies that both support or reject it are based on “a” sample from “a” population. As should be clear by now, we don’t know which people in the experimental or observed groups are genetically capable of digesting higher starch contents, higher animal fat, milk products and whose microbiome and oxidative phenotype agrees with a high-fat, low-carbohydrate diet.
The origin of the KD is the treatment for refractory (difficult to control and unresponsive to medication) epilepsy in children. Fasting had been used to control epilepsy since ancient times. However, it is not a sustainable method. In 1921, two physicians, Woodyatt and Wilder, published findings suggesting that diets containing very low amounts of carbohydrate and high amounts of fat mimicked the metabolic effects of starvation. Wilder treated patients at the Mayo Clinic where, in 1925, Peterman standardized the macronutrient composition of the KD: 1g per kg of body weight of protein, 10-15g of carbohydrate and the rest of the calories from fat (Wheless 2008).
By the end of the 1930s, the new anticonvulsant drug diphenylhydantoin was developed. The focus on epilepsy shifted from understanding the mechanisms of action of the KD to the development of new drugs. For decades the diet was forgotten even though about 20% of epileptic children are still refractory to anticonvulsants. Johns Hopkins hospital still offered the treatment.
KD attracted attention again in the late 1990s. Since then, it has been studied, sometimes successfully, to manage or treat a variety of neurological disorders and other pathologies, as well as obesity.
KD’s exact mechanism of action remains elusive. The metabolic changes result from glycogen depletion, very low levels of blood glucose and the breakdown of fatty acids into ketone bodies (acetoacetate, D-β-hydroxybutyrate (D-3-hydroxybutyrate), and acetone) in the liver, creating a state called “ketosis”. Under ketosis, the brain, which usually uses only glucose, can use ketone bodies as fuel.
Metabolic production of ketone bodies. From Ha & Bhagavan 2011
Alterations in intermediary metabolism during KD. From Hartman et al 2007
Alterations in the metabolism of excitatory amino acids and γ-aminobutyric acid (GABA) during KD. From Hartman et al 2007
These metabolic alterations happen in any animal on KD. The reasons why these alterations suppress convulsions and control symptoms of other neurological disorders are unknown. The first targets for investigation were the ketone bodies themselves, either through direct action or by affecting neurotransmitters metabolism (Harman et al 2007), and glycolysis restriction in favor of oxidative phosphorylation (Bough & Rho 2007). Other authors suggest that KD controls neurological disorders not though ketone bodies, but by a combination of mechanisms involving fat metabolism. Certain lipids, such as medium-chain fatty acids, have their own independent anticonvulsant effects. Anti-oxidant and anti-inflammatory properties are also candidates for the mechanisms observed during KD, as well as mitochondrial biogenesis and other epigenetic mechanisms. Finally, KD's anticonvulsant and neuroprotective action may also be related to its effect on the gut microbiome (Boison 2017, Bough et al 2006, Augustin et al 2018).
Why the KD controls or cures neuropsychiatric disorders has more to do with whatever is different in these patients than the mechanisms described above, which we know to be “associated” with the effects. The clues are mitochondrial defects or anomalies (Pei & Wallace 2018, Cabral-Costa & Kowaltowski 2020).
The polarization of opinions concerning KD is extreme in the exercise sciences and obesity studies. Either KB benefits athletes with no harm (or increase) to their performance or it’s incompatible with high performance (McSwiney et al 2019, Zinn et al 2017, McSwiney et al 2018, Greene et al 2018, Kang et al 2020). Either it is the ideal diet for obese patients or it is at least as effective as conventional diets and in a worst-case scenario, harmful.
A 2019 JAMA editorial (Joshi et al 2019) listed a number adverse effects from mild to severe, including the “keto flu,” an induction period of fatigue, weakness, and gastrointestinal disturbances, cardiac arrhythmias from selenium deficiency, nephrolithiasis, constipation, halitosis, muscle cramps, headaches, diarrhea, restricted growth, bone fractures, pancreatitis, and multiple vitamin and mineral deficiencies.
The Mayo Clinic “verdict” about the ketogenic diet is that it is harmful because it eliminates several important foods from a “healthy” diet, such as dairy products and whole grains.
The controversy persists.
All we can say now is that there are probably many people who will suffer adverse effects. Other people will thrive on the KD. The Innuit people, whose diet resembles the KD, have been around for a long time (Munch-Andersen et al 2012, Fumagalli et al 2015).
The other reason for the inconsistency of results is the confusion in nomenclature. Having "low-carbohydrate" says too little about a dietary program. What is the fiber content? What are the foods? What is exactly the macro-nutrient composition? Is it hypo or isocaloric? If hypo, how much so? With no standards in the protocol, we’re not even talking about the same thing (Trimboli et al 2020).
The key to understanding all these discrepancies is the new evidence concerning inter-individual variability due to genetic, epigenetic or gut microbiological factors. Besides being highly individualized, the gut microbiota has been implicated in a wide range of pathological and healthy conditions (Liu & Zhu 2018, Bliss &Whiteside 2018). Its biodiversity is also associated with the evolutionary diversification of dietary, digestive and metabolic constitution (Schonorr et al 2016). Finally, the gut microbiome is one of the chief participants and modulators of the inter-organ cross-talk (Martin et al 2018).
From Davidson et al 2018
Precision nutrition emerged in recent years to address these problems. At this point, it is an ambitious project involving mobile technology, data science, and on-ground portable diagnostics.
“Precision nutrition encompasses prevention and treatment strategies for optimizing nutritional status that consider individual variability in diet, lifestyle, environment, and genes by accurately determining an individual’s nutritional status.” (Srinivasan et al 2017)
If the infrastructure can be made affordable enough, dealing with major nutritional problems in different settings will be far more manageable.
A Few Takeaways
- There is no universal healthy diet or macronutrient composition. You must identify what is healthy for you.
- Tests are limited so trial and error and long-term observation are important. You probably know if you have major food intolerances or allergies. Writing down your reaction to changes in diet is helpful.
- If you feel you need to change your diet, try substituting or eliminating some items and observe how you react (if there is no improvement, re-include the item you excluded). Examples: Eliminate high glycemic index sugars (glucose, sucrose, maltodextrin, etc). Eliminate high glycemic index sugars and reduce as much as possible starchy foods, especially processed starch. Substitute grains for tubers and vice-versa. Eliminate dairy food for one month.
- If you need to lose fat, you need to reduce your total calorie intake. Try doing this by preferentially decreasing either fat or carbohydrate. If you have been unsuccessful with one approach, try the other.
- Take good care of your gut microbiota. It needs soluble and insoluble fibers, and as much fresh and unprocessed food as you can. Adding fermented foods to your diet or taking probiotic supplements may help, although this is still controversial.
References
- Augustin, Katrin, Aziza Khabbush, Sophie Williams, Simon Eaton, Michael Orford, J. Helen Cross, Simon JR Heales, Matthew C. Walker, and Robin SB Williams. "Mechanisms of action for the medium-chain triglyceride ketogenic diet in neurological and metabolic disorders." The Lancet Neurology 17, no. 1 (2018): 84-93.
- Boison, Detlev. "New insights into the mechanisms of the ketogenic diet." Current opinion in neurology 30, no. 2 (2017): 187.
- Bough, Kristopher J., and Jong M. Rho. "Anticonvulsant mechanisms of the ketogenic diet." Epilepsia 48, no. 1 (2007): 43-58.
- Bough, Kristopher J., Jonathon Wetherington, Bjørnar Hassel, Jean Francois Pare, Jeremy W. Gawryluk, James G. Greene, Renee Shaw, Yoland Smith, Jonathan D. Geiger, and Raymond J. Dingledine. "Mitochondrial biogenesis in the anticonvulsant mechanism of the ketogenic diet." Annals of Neurology: Official Journal of the American Neurological Association and the Child Neurology Society 60, no. 2 (2006): 223-235.
- Cabral-Costa, J. V., and A. J. Kowaltowski. "Neurological disorders and mitochondria." Molecular aspects of medicine (2020).
- Fumagalli, Matteo, Ida Moltke, Niels Grarup, Fernando Racimo, Peter Bjerregaard, Marit E. Jørgensen, Thorfinn S. Korneliussen et al. "Greenlandic Inuit show genetic signatures of diet and climate adaptation." Science 349, no. 6254 (2015): 1343-1347.
- Ha, Chung-Eun, and N. V. Bhagavan. Essentials of medical biochemistry: with clinical cases. Academic Press, 2011.
- Hartman, Adam L., Maciej Gasior, Eileen PG Vining, and Michael A. Rogawski. "The neuropharmacology of the ketogenic diet." Pediatric Neurology 36, no. 5 (2007): 281-292.
- Itan, Yuval, Bryony L. Jones, Catherine JE Ingram, Dallas M. Swallow, and Mark G. Thomas. "A worldwide correlation of lactase persistence phenotype and genotypes." BMC evolutionary biology 10, no. 1 (2010): 36.
- James, W. P. T., R. J. Johnson, J. R. Speakman, D. C. Wallace, G. Frühbeck, P. O. Iversen, and P. J. Stover. "Nutrition and its role in human evolution." Journal of internal medicine 285, no. 5 (2019): 533-549.
- Joshi, Shivam, Robert J. Ostfeld, and Michelle McMacken. "The ketogenic diet for obesity and diabetes—enthusiasm outpaces evidence." JAMA internal medicine 179, no. 9 (2019): 1163-1164.
- McSwiney, Fionn T., Lorna Doyle, Daniel J. Plews, and Caryn Zinn. "Impact Of Ketogenic Diet On Athletes: Current Insights." Open Access Journal of Sports Medicine 10 (2019): 171.
- Moreno, Cesar L., and Charles V. Mobbs. "Epigenetic mechanisms underlying lifespan and age-related effects of dietary restriction and the ketogenic diet." Molecular and cellular endocrinology 455 (2017): 33-40.
- Munch-Andersen, Thor, David B. Olsen, Hans Søndergaard, JensR Daugaard, Anette Bysted, Dirk L. Christensen, Bengt Saltin, and JørnW Helge. "Metabolic profile in two physically active Inuit groups consuming either a western or a traditional Inuit diet." International journal of circumpolar health 71, no. 1 (2012): 17342.
- Pei, Liming, and Douglas C. Wallace. "Mitochondrial etiology of neuropsychiatric disorders." Biological Psychiatry 83, no. 9 (2018): 722-730.
- Rangel, Adriano Henrique do Nascimento, Danielle Cavalcanti Sales, Stela Antas Urbano, José Geraldo Bezerra Galvão Júnior, Júlio César de Andrade Neto, and Cláudia de Souza Macêdo. "Lactose intolerance and cow's milk protein allergy." Food Science and Technology (Campinas) AHEAD (2016): 0-0.
- Rankin, Paula, Danielle Callanan, Kevin O’Brien, Gareth Davison, Emma J. Stevenson, and Emma Cockburn. "Can Milk Affect Recovery from Simulated Team-Sport Match Play?." Nutrients 12, no. 1 (2020): 112.
- Rankin, Paula, Michael J. Lawlor, Frank A. Hills, Phillip G. Bell, Emma J. Stevenson, and Emma Cockburn. "The effect of milk on recovery from repeat-sprint cycling in female team-sport athletes." Applied Physiology, Nutrition, and Metabolism 43, no. 2 (2018): 113-122
- Storhaug, Christian Løvold, Svein Kjetil Fosse, and Lars T. Fadnes. "Country, regional, and global estimates for lactose malabsorption in adults: a systematic review and meta-analysis." The Lancet Gastroenterology & Hepatology 2, no. 10 (2017): 738-746.
- Trimboli, Pierpaolo, Marco Castellana, Diego Bellido, and Felipe F. Casanueva. "Confusion in the nomenclature of ketogenic diets blurs evidence." Reviews in Endocrine and Metabolic Disorders (2020): 1-3.
- Wheless, James W. "History of the ketogenic diet." Epilepsia 49 (2008): 3-5.
- Zinn, Caryn, Matthew Wood, Mikki Williden, Simon Chatterton, and Ed Maunder. "Ketogenic diet benefits body composition and well-being but not performance in a pilot case study of New Zealand endurance athletes." Journal of the International Society of Sports Nutrition 14, no. 1 (2017): 22.
- McSwiney, Fionn T., Bruce Wardrop, Parker N. Hyde, Richard A. Lafountain, Jeff S. Volek, and Lorna Doyle. "Keto-adaptation enhances exercise performance and body composition responses to training in endurance athletes." Metabolism 81 (2018): 25-34.
- Greene, David A., Benjamin J. Varley, Timothy B. Hartwig, Phillip Chapman, and Michael Rigney. "A low-carbohydrate ketogenic diet reduces body mass without compromising performance in powerlifting and Olympic weightlifting athletes." The Journal of Strength & Conditioning Research 32, no. 12 (2018): 3373-3382.
- Kang, Jie, Nicholas A. Ratamess, Avery D. Faigenbaum, and Jill A. Bush. "Ergogenic Properties of Ketogenic Diets in Normal-Weight Individuals: A Systematic Review." Journal of the American College of Nutrition (2020): 1-11.
- Srinivasan, Balaji, Seoho Lee, David Erickson, and Saurabh Mehta. "Precision nutrition—Review of methods for point-of-care assessment of nutritional status." Current opinion in biotechnology 44 (2017): 103-108.
- Davidson, Gabrielle L., Amy C. Cooke, Crystal N. Johnson, and John L. Quinn. "The gut microbiome as a driver of individual variation in cognition and functional behavior." Philosophical Transactions of the Royal Society B: Biological Sciences 373, no. 1756 (2018): 20170286.
- Liu, Lu, and Gang Zhu. "Gut–brain axis and mood disorder." Frontiers in psychiatry 9 (2018): 223.
- Bliss, Edward S., and Eliza Whiteside. "The gut-brain axis, the human gut microbiota and their integration in the development of obesity." Frontiers in physiology 9 (2018): 900.
- Schnorr, Stephanie L., Krithivasan Sankaranarayanan, Cecil M. Lewis Jr, and Christina Warinner. "Insights into human evolution from ancient and contemporary microbiome studies." Current opinion in genetics & development 41 (2016): 14-26.
- Martin, Clair R., Vadim Osadchiy, Amir Kalani, and Emeran A. Mayer. "The brain-gut-microbiome axis." Cellular and molecular gastroenterology and hepatology 6, no. 2 (2018): 133-148.