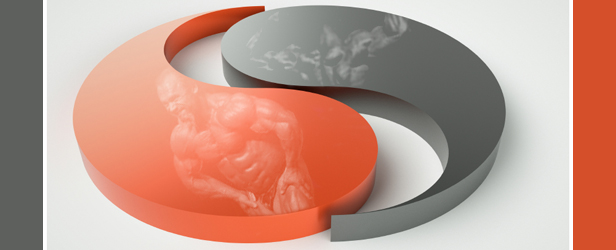
In part one of this article series, I introduced the complex role of arachidonic acid (ARA) in eicosanoid production, particularly in the context of using ARA supplementation to “trick” our bodies into growing by amplifying the inflammatory response to exercise. In Part two of this article series, we’ll keep digging. Does the available science support ARA as a quick n’ easy way to gain muscle mass?
ARA: Diet, Exercise and Supplementation
Does exercise use up arachidonic acid?
Because it repeatedly triggers inflammation, exercise training repeatedly draws upon ARA to produce eicosanoids (1, 2). Older lifters might take note especially, as ARA levels seem to decline with age (3, 4). [This may be due to due to reduced activity of the enzymes that convert dietary linoleic acid to ARA (5).]
Thus, training and advanced age are two considerations when forming a rationale for replenishing ARA. (Note: I haven’t found any research examining whether the reduction in ARA stored due to exercise is in any way limiting.)
ARA in our food?
ARA is found mainly in animal protein sources, which, of course, are not lacking in the diets of most bodybuilders (6–10). A 100-gram sample of beef, pork or turkey contains roughly 50 mg of ARA, with higher levels in fattier meats and whole eggs (11).
ARA part 2 supplements scott stevenson 092414
Diet or supplement to boost ARA levels?
Perhaps because the typical Western diet is already high in linoleic acid (LA), the parent compound for ARA, more dietary LA does not typically mean higher ARA levels in the body (12, 13). Similarly, higher LA intakes don’t have a clear association with overall inflammation (14) or health risks such as cardiovascular disease (15, 16), although this issue isn’t entirely resolved (17). This leaves supplemental ARA as the preferred means to boost tissue ARA stores (18).
How much ARA (and is it risky)?
Vegetarians have lower levels of ARA (19), and increasing meat consumption may increase ARA status (20). In a study of (untrained) Japanese men, both 240 mg and 720 mg of daily supplemental ARA increased ARA status dose dependently but had no effect on any measure of ARA metabolites [or inflammation (21)]. Roughly double this daily ARA supplemental dose (1500 mg for healthy young men weighing approximately 160 pounds) was enough to increase production of two pro-inflammatory eicosanoids [PGE2 and LTB4 (22)] but not clotting risk (23). On the other hand, in patients with cirrhotic liver disease, 2000 mg ARA per day may increase platelet clotting as well as ARA-derived eicosanoid levels (24).
So if ARA is to chronically affect downstream eicosanoid production in addition to ARA status (blood phospholipid levels), a supplemental dose of approximately 1500 mg per day would seem to be a reasonable starting point.
The Nitty Gritty: Does it Work?
As you can tell from the above, a black and white, cut and dry, pro-inflammatory boost from ARA has not been extensively studied. As far as using ARA to grow muscle, what about direct mechanistic and (almost) “real world” data (i.e. research with humans training for muscle size)?
Grow, cells, grow!
A study with (mouse) muscle cells in culture found that ARA does indeed stimulate dose-dependent increases in cell size and contractile protein, at least when compared to cells devoid of ARA (25). Fascinatingly, inhibiting cyclooxygenase (the COX2 isoform in particular) with non-steroidal anti-inflammatories (NSAIDs) prevented the anabolic effect of ARA, but giving more ARA meant that the NSAIDS were even more effective in preventing the cells from growing. In other words, the more “pro-inflammatory” ARA provided, the more devastating the inhibitory effect of the anti-inflammatories on cell growth.
To test the effects of eicosanoids themselves (versus the ARA they are derived from per se), the researchers also grew the muscle cells in an eicosanoid-rich (but ARA devoid) medium that had been produced previously by ARA-exposed cells (the ones that grew larger). One would expect that the ARA-derived eicosanoids would have stimulated growth, but quizzically, this was not the case. It seems that something about ARA (or its liberation ) per se (not simply the ARA-derived eicosanoids) may affect how developing muscle cells grow.
Another study (26) also used a mouse muscle cell model but employed mechanical stretch to trigger protein synthesis signaling and eicosanoid production (27). The stretch-induced mechanical signal for protein synthesis was neither enhanced by ARA nor was it inhibited by preventing eicosanoid synthesis. However, when they inhibited phospholipase A2 (PLA2), the signal for turning on protein synthesis signaling was reduced. You see, PLA2 mediates the liberation of ARA precursors from the cell membrane as well as the formation of lysophospholipids (28). This study suggests that neither ARA nor its eicosanoids seem to trigger protein synthesis, but that something about the release of ARA (via activity of the PLA2 enzyme) is important for anabolism.
When these stretched muscle cells were provided lysophosphatidylcholine—a particular lysophospholipid and product of PLA2 activity—this stimulated protein synthesis signaling in a dose-dependent manner (more lysophosphatidylcholine meant faster protein synthesis). This makes a certain amount of sense when one considers that lysophosphatidic acid (LPA) is a known signaling molecule for turning protein synthesis as a response to mechanical tension (29–31) and LPA can be derived from lysophosphatidylcholine [32 (which was anabolic in this study)] as well as phosphatidic acid, another dietary supplement with potential as an anabolic agent (33).
Essentially, this latter study using stretched muscle cells (26) tells us that there seems to be some regulation of muscle cell anabolism occurring at the site of liberating ARA (the PLA2 enzyme). Perhaps the processes upstream of making ARA (such as mechanical stimulation itself) are more important than the downstream actions of ARA per se.
Grow, muscle, grow?
Just in case you’re a bit confused, nowhere in this article have I come up with a clear picture of how or if ARA would serve as an anabolic dietary supplement. Indeed, a patent filed about 10 years ago contended that reducing ARA availability should be a feasible way to increase livestock carcass (muscle) yield, particularly by reducing the potential inflammation associated adverse health effects (34). Another patent awarded about the same time contends that supplementing with additional ARA is a viable means of increasing muscle mass (35).
More is probably not better
A “mega dosing” study of ARA in older rats [(36) fed 240 mg of ARA per kg per day or what would be about 39 grams of ARA per day for a 220-pound human (37)] led to oxidative stress and a reduction in type II muscle cell size. More is not always better.
Promising results?
In 2007, a study with humans (38) found that daily supplementation of 1000 mg ARA (previously resistance trained males performing seven weeks of training with four weight training sessions per week) had no benefit on body composition, muscle fiber size or strength gains. However, ARA increased cycling peak power (Wingate) compared to the placebo group (even though training did not involve cycling). ARA-derived prostaglandins PGF2a and PGE2, cortisol and testosterone were all unaffected by supplementation, but interleukin-6 (IL-6) was reduced at the end of training with ARA compared to the (unchanged) value in the placebo group. IL-6 is a myokine that aids in glycogen and fat mobilization during acute exercise (39, 40). However, it has both anti-inflammatory properties (41) and acute inflammatory action (41–43), so its role is complex. Chronic IL-6 elevation [e.g. due to adipose-derived IL-6 (40)] may indicate systemic inflammation (40, 43) and lowering it may reduce muscle breakdown (44).
A more recent collaboration (45) involving some of the authors of the above study shows more promise for ARA (although the study has not been published in a peer-reviewed journal). In recreationally trained men over eight weeks of resistance training, 1,500 mg of ARA per day promoted greater strength and cycling peak power gains as well as lean body mass gains (which did not occur in the placebo group). We’ll have to wait for further clarification of this study (other measurements have not been reported thus far) to appear in the peer-reviewed literature.
So what’s the verdict?
The evidence that I’ve been able to find doesn’t strongly suggest that “force feeding” Mother Nature supplemental ARA will have a predictable, mechanistically explainable effect on muscle growth. This is likely due to the complex intelligence underlying eicosanoid signaling (and muscle size). This intricate nature of eicosanoid signaling in directing muscle adaptation is highlighted in a series of studies published in the past decade where eicosanoid production was disrupted using non-steroidal anti-inflammatories (NSAIDs).
It’s been demonstrated that the NSAIDs ibuprofen and acetaminophen inhibit the production of the prostaglandin eicosanoids PGE2 and PGF2a (46) and impair muscle protein synthesis after resistance exercise, at least acutely (47). However, over the course of three months of resistance training, consuming these NSAIDs daily actually enhanced muscle growth (48).
To explain this bizarre finding, these researchers did a follow-up investigation (44) and discovered that the NSAID-consuming trainees had increased receptor density for PGF2a, which would have improved protein synthesis in response to exercise (49). Additionally, they speculate that by reducing PGE2 levels, which would have reduced catabolism (49), the NSAIDs reduced muscle protein breakdown, thus improving protein balance and muscle growth. In this case, it seems that Mother Nature took lemons (NSAIDs) and made lemonade (larger muscles)
Interestingly, this last finding fits well with recent studies of protein synthesis after supplementing with [generally anti-inflammatory (50)] fish oil. Eight weeks of supplementation with high w-3 fatty acid fish oil actually enhanced the anabolic responses to protein (amino acids) both with basal/post-absorptive (51) and elevated (52) blood insulin levels (e.g. after a meal).
Survey says?
We can glean the following general notions from this two-part article series on arachidonic acid:
Omega-3 fatty acids compete with ARA for deposition in the cell membrane as well as for enzymes of eicosanoid production.
ARA metabolites can be both pro- and anti-inflammatory.
Both w-3 and w-6 fatty acids may promote anabolism.
ARA may promote muscle cell growth, but this may not be mediated directly by its eicosanoids or other derivatives.
Impairing inflammation (with NSAIDS) may be anti-anabolic acutely, but chronic use may actually promote greater anabolic adaptations.
The evidence available so far isn’t detailed enough to dictate specifics of how, when or how much ARA one might supplement with to employ it as an anabolic agent (not to mention the mechanism of anabolic action). Perhaps there are individuals [e.g. vegetarians (19, 20)] who might be better responders to ARA, as is the case with creatine supplementation (53). Note that because the Western diet is typically replete with omega-6 fatty acids (54, 55), supplementing with large amounts of ARA warrants caution, especially in those concerned about inflammation [e.g., arthritis sufferers (56)] or with a propensity for blood clotting.
It’s good to see that the bodybuilding research lens has been redirected at ARA recently (45). To quote a television commercial from my childhood, I’m hoping that it won’t be long before we find out more about whether it’s “nice to fool Mother Nature” by using ARA to encourage muscle growth.
References
Andersson A, et al (2000) Fatty acid profile of skeletal muscle phospholipids in trained and untrained young men. Am J Physiol Endocrinol Metab 279(4):E744–51.
Helge JW, et al (2001) Training affects muscle phospholipid fatty acid composition in humans. J Appl Physiol (1985) 90(2):670–7.
Lynch MA, Voss KL (1994) Membrane arachidonic acid concentration correlates with age and induction of long-term potentiation in the dentate gyrus in the rat. Eur J Neurosci 6(6):1008–14.
Soderberg M, et al (1991) Fatty acid composition of brain phospholipids in aging and in Alzheimer’s disease. Lipids 26(6):421–5.
Maniongui C, et al (1993) Age-related changes in delta 6 and delta 5 desaturase activities in rat liver microsomes. Lipids 28(4):291–7.
Brill JB (1992) Pre-competition Dietary Practices of Competitive Male and Female Bodybuilders. Florida International University: Ann Arbor, pgs 117.
Hackett DA, et al (2013) Training practices and ergogenic aids used by male bodybuilders. J Strength Cond Res 27(6):1609–17.
Kleiner SM, et al (1990) Metabolic profiles, diet, and health practices of championship male and female bodybuilders. Journal of the American Dietetic Association 90(7):962–7.
Manore MM, et al (1993) Diet and exercise strategies of a world-class bodybuilder. Int J Sport Nutr 3(1):76–86.
Steen SN (1991) Pre-contest strategies of a male bodybuilder. Int J Sport Nutr 1(1):69–78.
Taber L, et al (1998) Assessment of the arachidonic acid content in foods commonly consumed in the American diet. Lipids 33(12):1151–7.
Rett BS, Whelan J (2011) Increasing dietary linoleic acid does not increase tissue arachidonic acid content in adults consuming Western-type diets: A systematic review. Nutr Metab (Lond) 8:36.
Adam O, et al (2008) Impact of linoleic acid intake on arachidonic acid formation and eicosanoid biosynthesis in humans. Prostaglandins Leukot Essent Fatty Acids 79(3–5):177–81.
Fritsche KL (2008) Too much linoleic acid promotes inflammation—doesn’t it? Prostaglandins, Leukotrienes and Essential Fatty Acids 79(3–5):173–5.
Harris WS (2008) Linoleic acid and coronary heart disease. Prostaglandins, Leukotrienes and Essential Fatty Acids 79(3–5):169–171.
Harris WS, et al (2009) Omega-6 fatty acids and risk for cardiovascular disease: A science advisory from the American Heart Association Nutrition Subcommittee of the Council on Nutrition, Physical Activity, and Metabolism; Council on Cardiovascular Nursing; and Council on Epidemiology and Prevention. Circulation 119(6):902–7.
Tribole E (2009) What happened to do no harm? The issue of dietary omega-6 fatty acids. Prostaglandins, Leukotrienes and Essential Fatty Acids 80(1):78–9.
Whelan J, et al (1993) Dietary arachidonate enhances tissue arachidonate levels and eicosanoid production in Syrian hamsters. J Nutr 123(12):2174–85.
Phinney SD, et al (1990) Reduced arachidonate in serum phospholipids and cholesteryl esters associated with vegetarian diets in humans. The American Journal of Clinical Nutrition 51(3):385–92.
Sinclair AJ, et al (1994) Diets rich in lean beef increase arachidonic acid and long-chain ω3 polyunsaturated fatty acid levels in plasma phospholipids. Lipids 29(5):337–43.
Kakutani S, et al (2011) Supplementation of arachidonic acid-enriched oil increases arachidonic acid contents in plasma phospholipids, but does not increase their metabolites and clinical parameters in Japanese healthy elderly individuals: A randomized controlled study. Lipids Health Dis 10:241.
Kelley DS, et al (1998) Arachidonic acid supplementation enhances synthesis of eicosanoids without suppressing immune functions in young healthy men. Lipids 33(2): 125–30.
Nelson GJ, et al (1997) The effect of dietary arachidonic acid on platelet function, platelet fatty acid composition, and blood coagulation in humans. Lipids 32(4):421–5.
Pantaleo P, et al (2004) Effects of dietary supplementation with arachidonic acid on platelet and renal function in patients with cirrhosis. Clin Sci (Lond) 106(1):27–34.
Markworth JF, Cameron-Smith D (2013) Arachidonic acid supplementation enhances in vitro skeletal muscle cell growth via a COX-2-dependent pathway. Am J Physiol Cell Physiol 304(1):C56–67.
Burkholder TJ (2009) Stretch-induced ERK2 phosphorylation requires PLA2 activity in skeletal myotubes. Biochemical and Biophysical Research Communications 386(1):60–4.
Vandenburgh HH, et al (1990) Stretch-induced prostaglandins and protein turnover in cultured skeletal muscle. Am J Physiol 259(2Pt1):C232–40.
Six DA, Dennis EA (2000) The expanding superfamily of phospholipase A(2) enzymes: classification and characterization. Biochim Biophys Acta 1488(1–2):1–19.
Winter JN, et al (2010) Phosphatidic acid mediates activation of mTORC1 through the ERK signaling pathway. Am J Physiol Cell Physiol 299(2):C335–44.
Kam Y, Exton JH (2004) Role of phospholipase D1 in the regulation of mTOR activity by lysophosphatidic acid. Faseb J 18(2):311–9.
Hornberger TA, et al (2006) The role of phospholipase D and phosphatidic acid in the mechanical activation of mTOR signaling in skeletal muscle. Proceedings of the National Academy of Sciences of the United States of America 103(12):4741–6.
Liscovitch M, et al (1993) Phospholipase D-mediated hydrolysis of phosphatidylcholine: role in cell signaling. J Lipid Mediat 8(3):177–82.
Joy JM, et al (2013) Phosphatidic acid supplementation increases skeletal muscle hypertrophy and strength. Journal of the International Society of Sports Nutrition 10(Supp1):P13.
Cook M, et al. Method for improving body weight uniformity and increasing carcass yield in animals. U.S.P.a.T. Office. At:http://www.google.com/patents/US20050123586.
Llewellyn W. Use of arachidonic acid as a method of increasing skeletal muscle mass. U.S.P.a.T. Office. At: http://www.google.com/patents/US20040102519.
Inoue T, et al (2014) Effects of chronic administration of arachidonic acid on lipid profiles and morphology in the skeletal muscles of aged rats. Prostaglandins Leukot Essent Fatty Acids.
Sharma V, McNeill JH (2009), To scale or not to scale: the principles of dose extrapolation. Br J Pharmacol 157(6):907–21.
Roberts MD, et al (20047) Effects of arachidonic acid supplementation on training adaptations in resistance-trained males. J Int Soc Sports Nutr 4:21.
Glund S, et al (2007) Interleukin-6 Directly Increases Glucose Metabolism in Resting Human Skeletal Muscle. Diabetes 56(6):1630–7.
Keller P, et al (2003) Interleukin-6 production by contracting human skeletal muscle: autocrine regulation by IL-6. Biochem Biophys Res Commun 310(2):550–4.
Pratesi A, et al (2013) Skeletal muscle: An endocrine organ. Clin Cases Miner Bone Metab 10(1):11–4.
Louis E, et al (2007) Time course of proteolytic, cytokine, and myostatin gene expression after acute exercise in human skeletal muscle. J Appl Physiol (1985) 103(5):1744–51.
Ostrowski K, et al (1999) Pro- and anti-inflammatory cytokine balance in strenuous exercise in humans. The Journal of Physiology 515(1):287–91.
Trappe TA, et al (2013) Prostaglandin and myokine involvement in the cyclooxygenase-inhibiting drug enhancement of skeletal muscle adaptations to resistance exercise in older adults. Am J Physiol Regul Integr Comp Physiol 304(3):R198–205.
Ormes J, et al (2014) Effects of Arachidonic Acid Supplementation on Skeletal Muscle Mass, Strength, and Power. NSCA National Conference 2014, Las Vegas, July 9–12.
Trappe TA, et al (2001) Skeletal muscle PGF(2)(alpha) and PGE(2) in response to eccentric resistance exercise: influence of ibuprofen acetaminophen. The Journal of Clinical Endocrinology and Metabolism 86(10):5067–70.
Trappe TA, et al (2002) Effect of ibuprofen and acetaminophen on post-exercise muscle protein synthesis. American Journal of Physiology: Endocrinology and Metabolism 282(3):E551–6.
Trappe TA, et al (2011) Influence of acetaminophen and ibuprofen on skeletal muscle adaptations to resistance exercise in older adults. American Journal of Physiology: Regulatory, Integrative and Comparative Physiology 300(3):R655–62.
Rodemann HP, Goldberg AL (1982) Arachidonic acid, prostaglandin E2 and F2 alpha influence rates of protein turnover in skeletal and cardiac muscle. The Journal of Biological Chemistry 257(4):1632–8.
Calder PC (2010) Omega-3 fatty acids and inflammatory processes. Nutrients 2(3):355–74.
Smith GI, et al (2011) Dietary omega-3 fatty acid supplementation increases the rate of muscle protein synthesis in older adults: a randomized controlled trial. The American Journal of Clinical Nutrition 93(2):402–12.
Smith GI, et al (2011) Omega-3 polyunsaturated fatty acids augment the muscle protein anabolic response to hyperinsulinaemia-hyperaminoacidaemia in healthy young and middle-aged men and women. Clinical Science 121(6):267–78.
Burke DG, et al (2003) Effect of creatine and weight training on muscle creatine and performance in vegetarians. Med Sci Sports Exerc 35(11):1946–55.
Simopoulos AP, et al (1999) Workshop on the essentiality of and recommended dietary intakes for omega-6 and omega-3 fatty acids. Journal of the American College of Nutrition 18(5):487–9.
Erasmus U (1993) Fats that heal, fats that kill: The complete guide to fats, oils, cholesterol, and human health. Rev. updated and expanded ed. Burnaby, BC, Canada: Alive Books, pgs 456.
Adam O, et al (2003) Anti-inflammatory effects of a low arachidonic acid diet and fish oil in patients with rheumatoid arthritis. Rheumatology International 23(1):27–36.
2 Comments